Research at the Bionics, Systems and Control Laboratory at Syracuse University focuses on the modeling, control design and analysis, and experimental implementation of human-robot interactions that arise during rehabilitative tasks such exoskeletal assisted walking, motorized cycling, neuromuscular control, and operant conditioning of lower-limb muscles. Abstracts of the ongoing projects are described below:
Hybrid Lower-Limb Exoskeleton Nonlinear and Adaptive Control
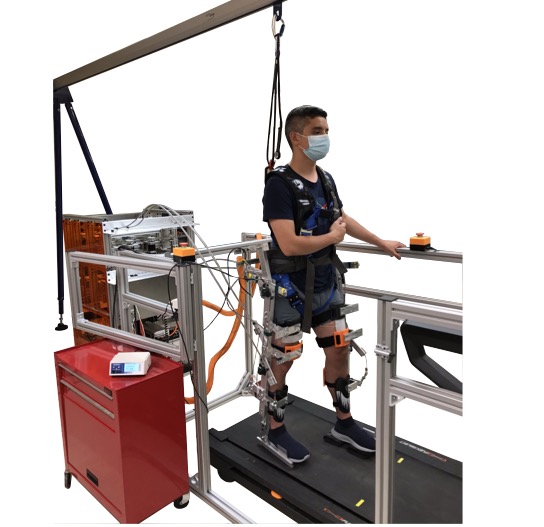
Wearable exoskeletons and neuromuscular control have the potential to improve locomotor ability and muscle capacity in individuals with movement disorders following a neurologic injury. This project aims to advance the gait performance of lower limb powered exoskeletons leveraging switched and hybrid systems theory to design robust and adaptive algorithms. The control methods coordinate the activation of muscles via FES and a powered exoskeleton during the gait cycle while guaranteeing stability and tracking performance using Lyapunov-based methods. Switching kinematic and force controllers are developed to provide power assist adjusting the stiffness and damping of legs. Adaptive-based controllers are developed to estimate uncertain parameters of the human-robot dynamics to improve gait control. Experimental demonstrations are conducted using a cable-driven exoskeleton and surface FES for treadmill walking.
Closed-loop Control for Motorized FES-Cycling
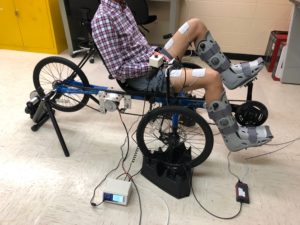
Functional electrical stimulation (FES)-induced cycling is a rehabilitation strategy that activates lower-limb muscles to achieve coordinated pedaling in individuals with movement disorders. An electric motor is included in-the-loop assisting the rider as needed to prolong exercise duration and mitigate muscle fatigue. Cycling objectives include cadence (speed) and torque tracking in which muscles are activated alongside the electric motor. The control design strategies include learning-based methods to cope with uncertainty in the system, finite-time control algorithms to ensure convergence of estimates and switching approaches to share the control between muscles and machine. Experimental demonstrations are conducted using a stationary recumbent motorized cycle.
Closed-loop Control and Operant Conditioning of Soleus Muscle
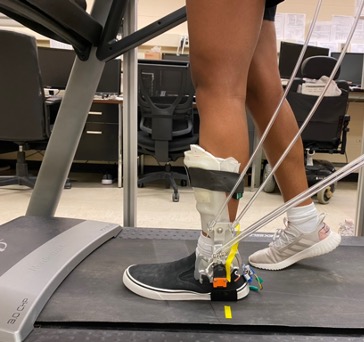
Stroke survivors experience muscle weakness and low weight-bearing capacity that impair locomotion. The ankle joint and soleus muscle are critically important during walking, as they store mechanical energy throughout the stance phase, leading to large plantarflexor activation at push-off for propulsion. However, plantarflexor activity and propulsive force are diminished in the paretic leg. This project focuses on the closed-loop control of a wearable device to control the ankle kinematics in mid-late stance phase and characterize the changes in soleus EMG. The control methods adjust the soleus stimuli continuously and reliably for developing a novel operant conditioning protocol to enhance ankle power and propulsion, which can improve gait after a stroke.